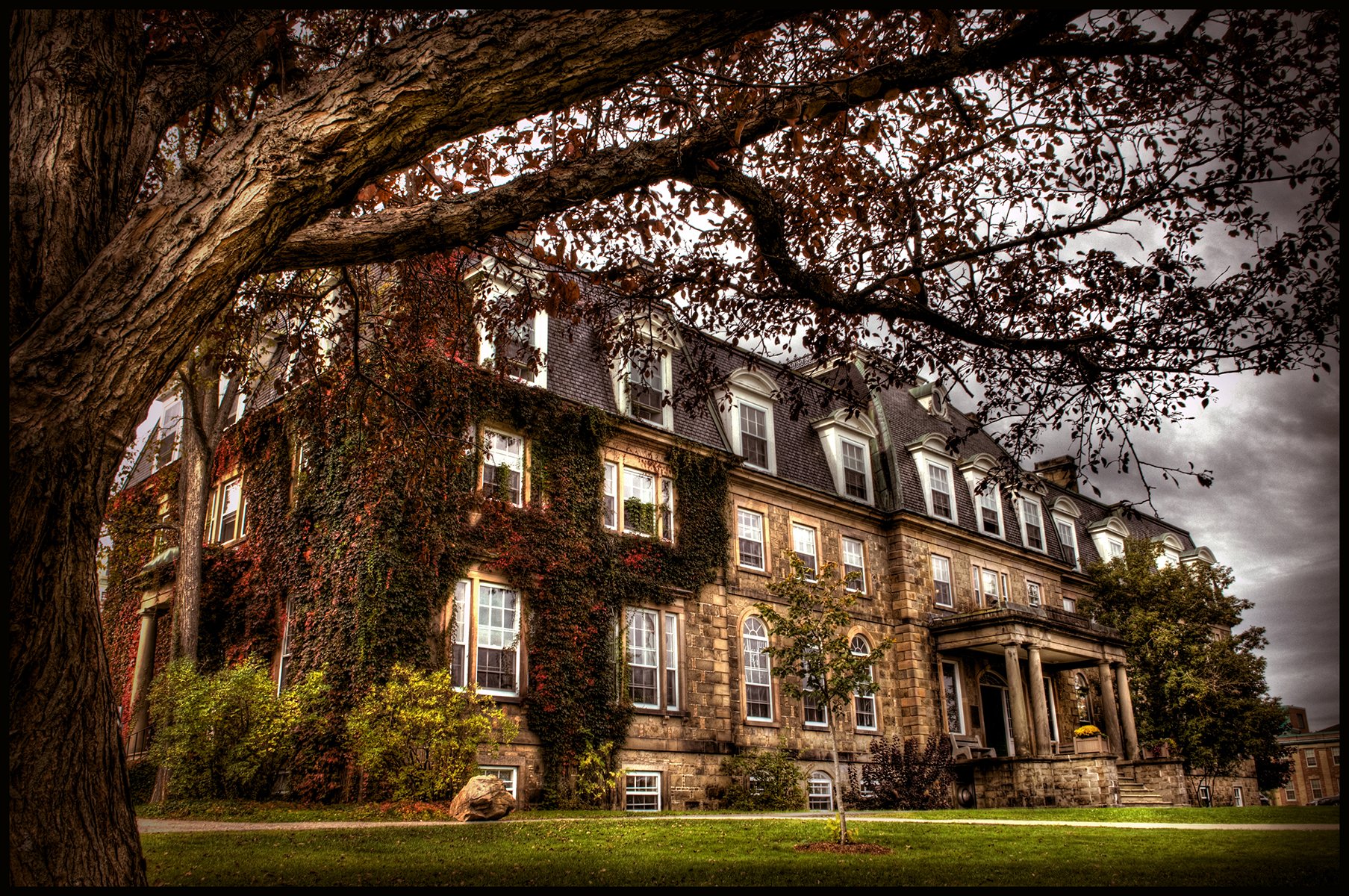
Research Projects
Rubidium spectrometer used to lock our 780 nm master laser.
Tunable Frequency-Offset-Locked Laser System for Matter-Wave Interferometry
We are developing fast opto-electronics for stabilizing a diode laser using a frequency-offset locking scheme. Our 1560 nm diode laser is slaved to an ultra-stable master laser operating at 780 nm by frequency-doubling the slave laser and measuring its optical beat note with the master on a high-speed photodiode. This RF beat note is fed through a broadband variable divider and a frequency-to-voltage converter with a high degree of linearity. An analog proportional-integrator circuit then uses the corresponding voltage signal to control the slave laser’s frequency with a tunable offset in the range of ~100 MHz to a few GHz. This locking system is deployed on a laser-cooling experiment to realize a quantum gravimeter based on matter-wave interferometry.
See our Poster Presentation for more details.
Microwave synthesizer for laser cooling and atom interferometry.
Ultra-Low Phase Noise Microwave Synthesizer for Quantum Sensing with Cold Atoms
Ultra-low phase noise microwave synthesizers are critical for achieving high-precision quantum sensors based on cold-atom interferometry. Our synthesizer is designed for both laser cooling rubidium-87 atoms and inducing ground state Raman transitions that function as the atom-optical pulses in our interferometer. During these pulses, the phase of the Raman laser is directly imprinted on the atomic wavefunction. Thus, for sensitive quantum measurements, a very low noise is desired for the microwave signal phase that is transferred to the atoms. Our synthesizer design generates two independent microwave signals: one at 6.6 GHz that acts as a repump frequency for laser cooling, and one at 6.834 GHz — the hyperfine splitting in rubidium. Both signals are derived from an ultra-stable 100 MHz ovenized crystal oscillator and a phase-locked dielectric resonator oscillator operating at 3.35 GHz. The two microwave signals are combined and sent to an electro-optic phase modulator to generate the desired optical frequencies in our 780 nm laser system. Preliminary measurements of the microwave power spectral density at 6.7 GHz yield a phase noise of −85 dB·rad²/Hz at an offset of 10 Hz. For a Mach-Zehnder type atom interferometer with a free fall time of T = 100 ms, we estimate a root mean squared phase noise of 135 mrad—corresponding to less than 1E-9 g per shot in a quantum gravimeter.
See our Poster Presentation for more details.
Compact quartz cell and optics for cold rubidium source.
Ultracold Rubidium Source
Cold atoms are ideal for high-accuracy inertial sensing using atom interferometry. The dominant systematic effect in atom interferometers using thermal clouds is due to the expansion of the atoms in the excitation beam (i.e. wavefront distortion). Ultracold sources are therefore essential for minimizing this effect and realizing accurate inertial sensors.
We are contructing an ultracold source of rubidium-87 atoms for a high-accuracy quantum gravimeter. We use a multi-stage cooling process involving a 2D+ magneto-optical trap (MOT), a 3D MOT, and an off-resonant optical dipole trap (ODT). A compact quartz cell with an integrated rubidium dispensor facilitates the production of a cold atomic beam using a 2D+ geometry with custom-designed magneto-optical hardware capable of fast magnetic field switching. The atomic beam loads a 3D MOT in a titanium science chamber with lower background pressure—ensuring extended trap lifetimes and interrogation times. After an initial cooling and compression stage in the 3D MOT, the atoms are loaded into an ODT where their temperature is further reduced using a combination of evaporative and delta-kicked cooling techniques.
Non-magnetic UHV system for quantum sensing.
Vectorial Quantum Sensing Platform
We are constructing a vectorial quantum sensing platform to develop a new class of multi-axis atom interferometry. This platform is designed to provide high-accuracy measurements of local time (i.e., an atomic clock), and vector quantities such as acceleration, rotation rate, and magnetic field.
Most cold-atom-based sensors can measure only single components of 3D vector quantities like acceleration. Our architecture utilizes three mutually-orthogonal lasers—providing full vector sensitivity in a single device. This vectorial quantum sensor will require a new type of “atom optic” involving multidimensional atomic diffraction. This will enable new 2D and 3D geometries of matter-wave interferometers that are sensitive to all components of the acceleration and rotation vectors—providing a full inertial base for positioning and navigation applications. The same beam geometry can be harnessed to measure the full magnetic field vector. Finally, accurate measurements of local time can be realized a Ramsey-type atomic clock in a fountain geometry. Combining these capabilities in a single compact device would form the basis of a unique multifunctional instrument with several applications. For instance, the intrinsically 3D nature of this architecture opens new possibilities for ultra-high accuracy atomic gyroscopes—making it directly applicable to geophysicists in rotational seismology, and several civilian, military, and space industries utilizing state-of-the-art inertial navigation systems.
BSc Honours Projects
Sam Moir (2023) - A Fiber-Coupled Optical Delivery System for a 2D+ Magneto-Optical Trap
Kamal Shalaby (2022) - Laser Frequency Stabilization using a Microcontroller